Development of Fundamental Technology
Pressure coring and analysis technology
(Pressure-core Nondestructive Analysis Tools PNATs)
In order to gain an understanding of the amount of gas that can be produced from methane hydrate-bearing layers, we must first have detailed knowledge of the physical properties of methane hydrate itself, and the state and physical properties of methane hydrate-bearing layers.
Since methane hydrate is stable at low temperature and high pressure conditions, investigating its state and measuring its physical properties requires us to fabricate, from scratch, a special experimental system capable of observing and analyzing targets with variations in a low-temperature and high-pressure environment.
A comprehensive system to analyze methane hydrate sediment cores could be developed to gain an understanding of the geophysical and geo-mechanical properties of methane hydrate reservoirs. The analyses and studies of the pressured cores will be carried out at AIST Hokkaido Center in Japan. [1]-[4]
AIST introduced Pressure-core Nondestructive Analysis Tools (PNATs), in which pressure cores are handled without depressurizing the pore pressure until core samples have been set up.
The PNATs can provide essential reservoir parameters such as permeability, hydrate saturation, X-ray CT images, p-wave responses, mechanical properties, and so on, under full pressurized operation. The following advanced facility and testing tools can be used to conduct fully pressurized operations and provide essential reservoir parameters (Fig.1, Fig.2).
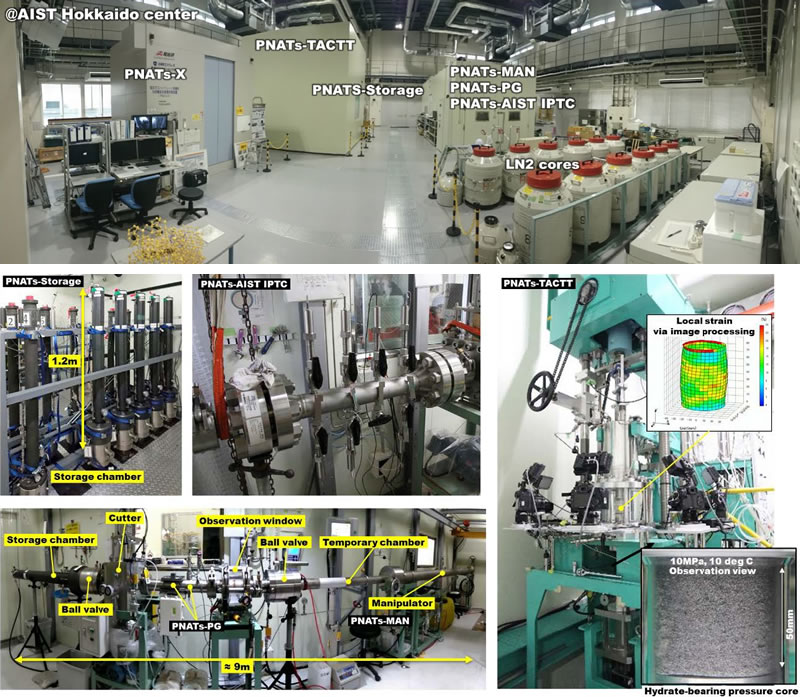
Fig.1 Pressure-core Nondestructive Analysis Tools,:PNATs
The upper left photograph shows the storage chamber for pressure cores. As shown therein, one storage chamber can accommodate cores of up to 1.2 meters in height. The upper right photograph shows testing apparatus used to measure mechanical properties and permeability. To enable pressure cores to be visualized, acrylic cells are used so that it is possible to observe the core as shown in the photograph.
Shown in the photograph below is equipment used to cut pressure cores to be used for various measurements, which also enables elastic waves to be measured.
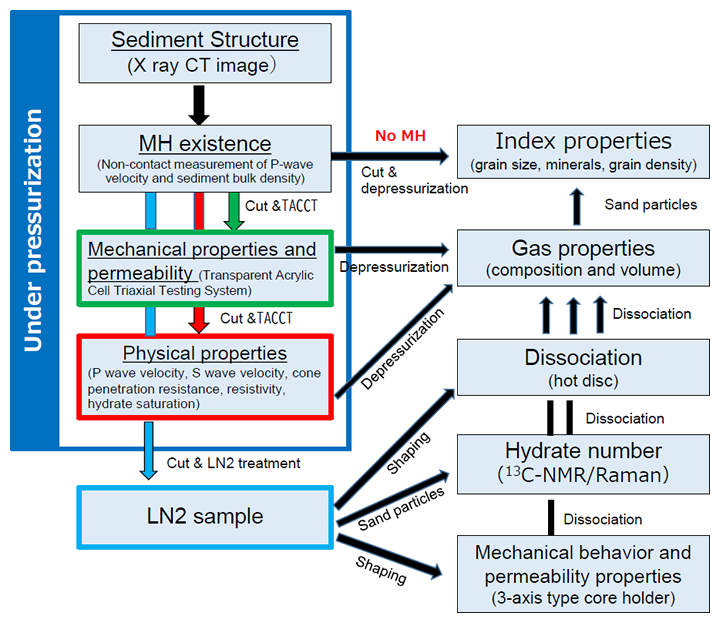
Fig.2 Flowchart of PNAT’s
Reference
- [1] Yoneda, J., Masui, A., Tenma, N., Nagao, J., 2013. Triaxial testing system for pressure core analysis using image processing technique. Rev. Sci. Instrum. 84, 114503.
- [2] Yoneda, J., Masui, A., Konno, Y., Jin, Y., Egawa, K., Kida, M., Ito, T., Nagao, J., Tenma, N., 2015, Mechanical behavior of hydrate-bearing pressure core sediments visualized under tri-axial compression. Marine and Petroleum Geology, 66, 451–459.
- [3] Jin, Y., Konno, Y., Yoneda, J., Kida, M., Nagao, J., 2016, In situ methane hydrate morphology investigation: natural gas hydrate-bearing sediment recovered from the eastern Nankai Trough area. Energy Fuels 30 (7), 5547–5554.
- [4] Yoneda, J., Masui, A., Konno, Y., Jin, Y., Kida, M., Katagiri, J., Nagao, J., Tenma, N., 2017, Pressure-core-based reservoir characterization for geomechanics: Insights from gas hydrate drilling during 2012-2013 at the eastern Nankai Trough, Marine and Petroleum Geology, 86, 1-16.
Methane hydrate production simulator and depressurization method
Data acquired from the state and physical properties analysis of methane hydrate-bearing layers were loaded into the “MH21-HYDRES” methane hydrate production simulator developed in Japan. A key characteristic of MH21-HYDRES is that it has been specifically designed for use in the development of methane hydrate resources. The main differences from ordinary reservoir simulators designed for the exploitation of conventional oil and gas are as follows:
- Target phases: gas, water, methane hydrate, and ice
- Target components: methane, nitrogen, carbon dioxide, water, methanol, and salt
- Able to simulate dissociation / formation of methane hydrate and dissolution / formation of ice based on kinetics equations
- Able to simulate the exothermic and endothermic behaviors associated with both dissociation / formation of methane hydrate, and dissolution / formation of ice
- Able to shift the three-phase equilibration curve of methane hydrate- methane-water (ice) depending on the concentrations of methanol or salt
- Able to modify the solubility of methane into the water phase by taking into account the salinity concentration
- Possible calculations of the mixed hydrate phases of methane, nitrogen, and carbon dioxide as well as methane hydrate
- Introducing the effective permeability and relative permeability as a function of methane hydrate saturation / ice
- Able to handle the analyses of the productivity and the production behavior in various basic production methods and their combinations such as depressurization, thermal stimulation, thermal flooding, inhibitor injection, and nitrogen injection
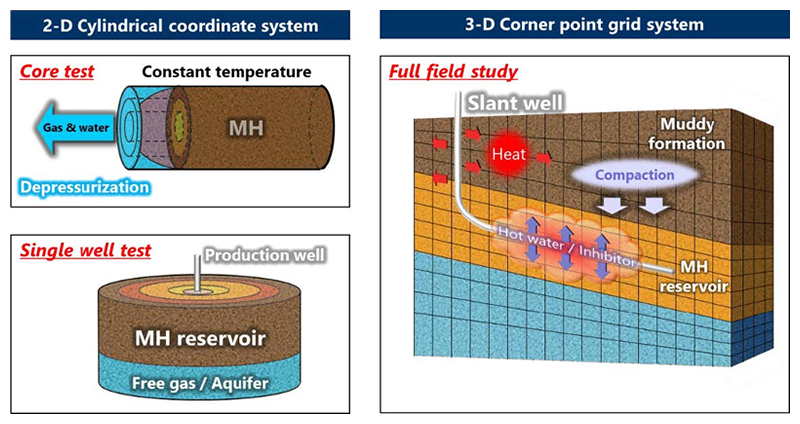
Fig.3 Outline of MH21-HYDRES
Since MH21-HYDRES allows for analysis of two-dimensional cylindrical coordinate system model (left figure) and three-dimensional Cartesian coordinate system model (right figure), it is used for the evaluation of laboratory tests (core tests) as well as field tests (single well tests, full field study) as shown in the above figure.
MH21-HYDRES is highly evaluated worldwide for its capability to simulate new production methods from the more than 10 production methods currently available based on the development-level scope and time scales. The production methods include the depressurization method, thermal stimulation method, inhibitor injection method, heterogeneous gas injection method, and a combination of the above.
Using MH21-HYDRES, we searched for a suitable method of methane hydrate production utilizing variations of methane hydrate occurrences and production methods. The optimum production method derived from the above study was the one based on the depressurization method.
In addition, MH21-HYDRES also has considerable records that have been used in behavior prediction, and test analysis for various real fields [1-5]. These simulation results contributed to the success of the first and second production tests carried out offshore of Japan. Furthermore, MH21-HYDRES has been used in a wide range of applications such as production behavior prediction for economic estimation, and examination of new production methods [6-7].
Reference
- [1] M. Kurihara, A. Sato, H. Ouchi, Y. Ohbuchi, Y. Masuda, H. Narita, T. Ebinuma, and T. Fujii, 2009: “Examination on gas producibility from Eastern Nankai Trough methane hydrate resources”, Journal of the Japanese Association for Petroleum Technology, Vol. 74, No. 4, pp. 311-324
- [2] H. Ouchi, M. Kurihara, A. Sato, Y. Masuda, H. Narita, T. Ebinuma, T. Saeki, T. Fujii, T. Kobayashi, and N. Shimoda, 2010: “Construction of 3 dimensional methane hydrate reservoir model in Eastern Nankai Trough and prediction of production test performance”, Journal of the Japanese Association for Petroleum Technology, Vol. 75, No.1, pp. 72-83
- [3] M. Kurihara, A. Sato, K. Funatsu, H. Ouchi, Y. Masuda, H. Narita, and T. Collett, 2011: “Analysis of formation pressure test results in the Mount Elbert methane hydrate reservoir through numerical simulation”, Marine and Petroleum Geology, Vol 28, Issue 2, Feb 2011, pp. 502-516
- [4] M. Kurihara, A. Sato, K. Funatsu, H. Ouchi, K. Yamamoto, M. Numasawa, T. Ebinuma, H. Narita, Y. Masuda, S. Dallimore, F. Wright, and D. Ashford, 2010: “Analysis of Production Data for 2007/2008 Mallik Gas Hydrate Production Tests in Canada”, SPE International Oil and Gas Conference and Exhibition in China, 8-10 June, Beijing, China, SPE 132155
- [5] Y. Konno, T. Fujii, A. Sato, K. Akamine, M. Naiki, Y. Masuda, K. Yamamoto, and J. Nagao, 2017: “Key Findings of the World's First Offshore Methane Hydrate Production Test off the Coast of Japan: Toward Future Commercial Production”, Energy & Fuels, Vol. 31, No.3, pp. 2607-2616
- [6] Y. Konno, Y. Masuda, Y. Hariguchi, M. Kurihara, and H. Ouchi, 2010: “Key Factors for Depressurization-Induced Gas Production from Oceanic Methane Hydrates”, Energy Fuels 24, pp. 1736-1744
- [7] Y. Konno, Y. Masuda, K. Akamine, M. Naiki, and J. Nagao, 2016: “Sustainable gas production from methane hydrate reservoirs by the cyclic depressurization method”, Energy Conversion and Management, Vol. 108, pp. 439-445